Speaking about HIIT training, work and recovery phases can be set with the help of two fundamental parameters: critical velocity (CV) and D’ (phenomenological equivalents to critical power (CP) and W’). Thus, CV defines the maximal exercise intensity at which a metabolic steady state can be attained during running exercise (Jones et al. 2019; Nixon et al. 2021). D’, on the other hand, is better defined as a work capacity (running distance) above CV (Fig. 1). Interestingly, the greater the amount of D’ is used the faster it recovers. This is because the reconstitution of D’ (or W’) follows a bi-exponential trajectory (Fig. 2), with the initial fast component (τFC) followed by a “sluggish” slow component (τSC; Caen et al. 2021; Chorley et al. 2021).
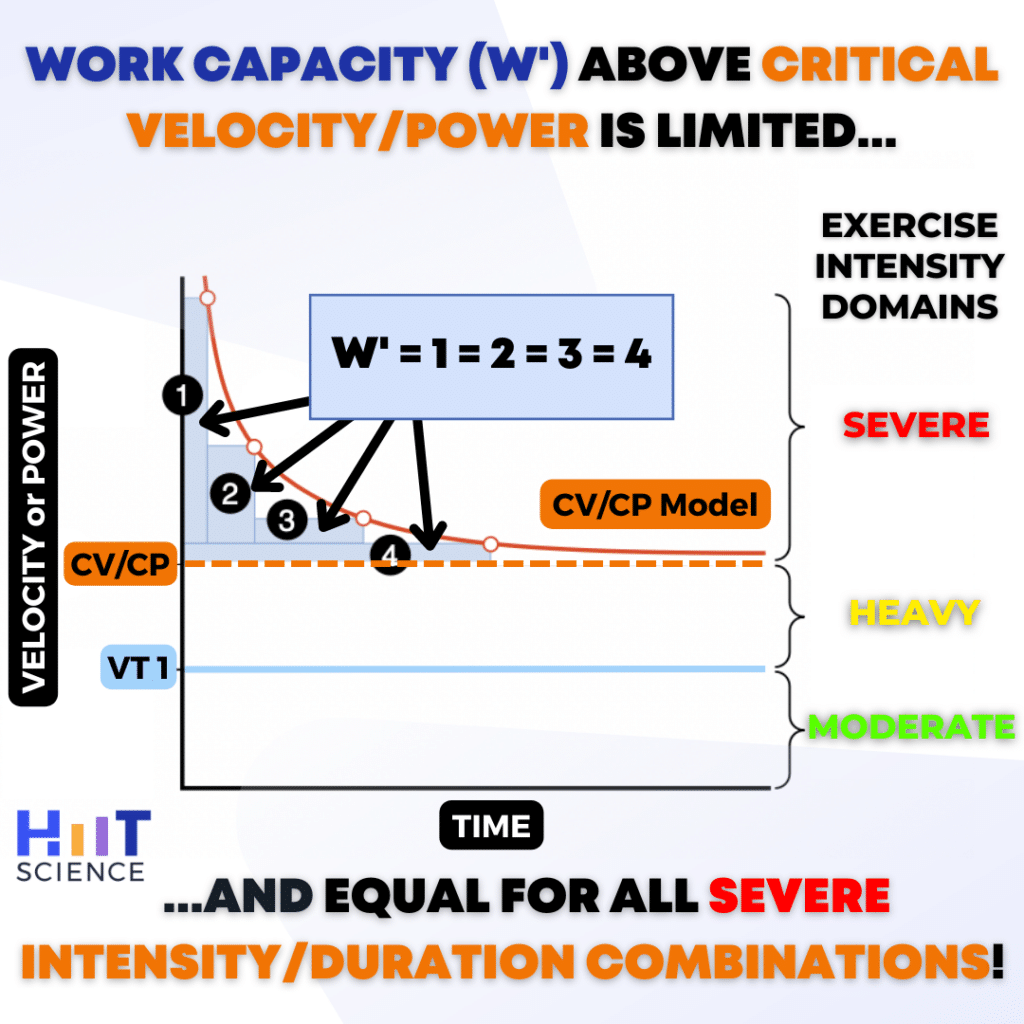
Figure 1. Critical velocity/power (CV/CP) and distance/work capacity (D’/W’). CP delimits the severe (metabolically unstable domain). W’ concept is illustrated using the boxes numbered 1 through 4, which all show different usages of W’ across varying intensity/duration combinations in the severe domain. Despite the different intensity/duration combinations, all W’ boxes display the same total finite area. VT1: ventilatory threshold 1 (aerobic threshold). Adapted from Jones et al. 2010.
Hence, a HIIT protocol that appreciates the kinetics of W’ recovery can allow more time at intensities close to VO2max to be achieved during a training session (Ferguson et al. 2010; Skiba et al. 2014; Caen et al. 2019; Sreedhara et al. 2020; do Nascimento Salvador et al. 2021; Lievens et al. 2021).
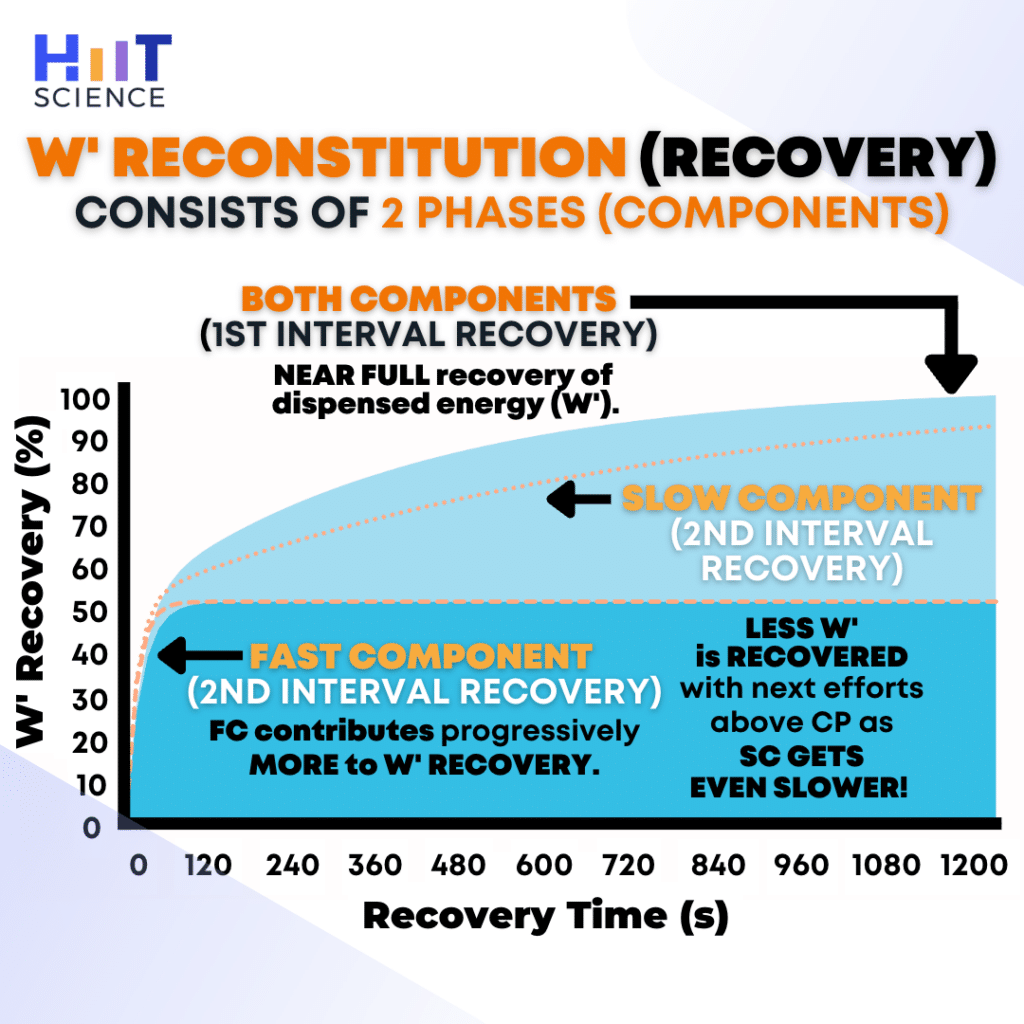
Better sooner than later – the rationale behind HIDIT
Given the reconstitution behaviour of D’ and knowing that starting with long (3-5 minutes) intervals causes a higher rise of VO2 compared to short (< 60 seconds) intervals (Millet et al. 2003; Jones et al. 2008), we thought that a decreasing high-intensity interval training protocol (HIDIT; Fig. 3) could exploit those advantages to allow a longer total workout time close to VO2max compared to classic short and long HIIT formats.
Practically speaking, HIDIT starts with long intervals that allow a faster rise of VO2, known as the “priming effect” (Fig. 4; Burnley et al. 2002; Jones et al. 2008) and more extensive use of D’ at the beginning of the high-intensity set. The successive decreasing intervals rely more on the reconstitution of D’ itself since efficient management of this work capacity is the key component to maintaining a near-maximal VO2 for longer.
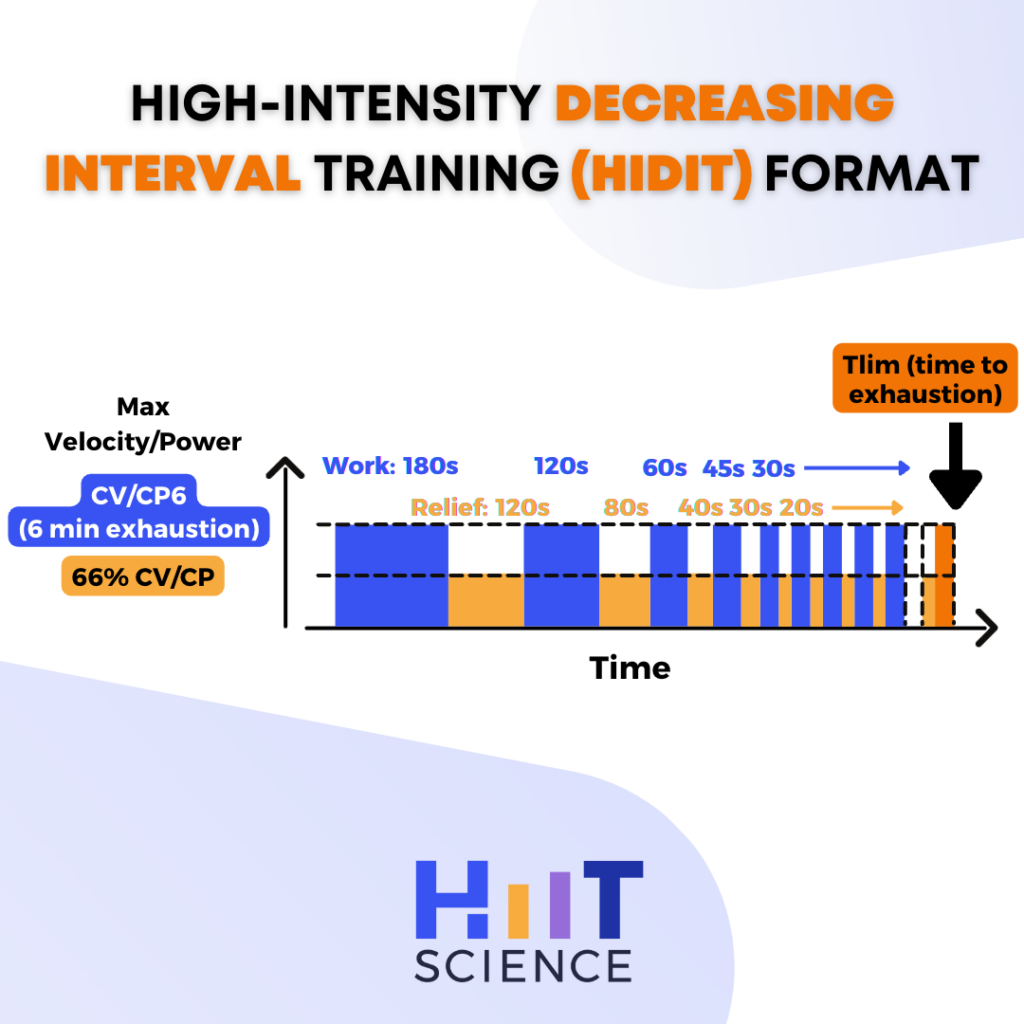
From our previous work with cyclists (Vaccari et al. 2020), we found that HIDIT had longer times above 90% of VO2max (T90%VO2max) than the short and long HIIT protocols. This time we wanted to test this hypothesis in runners.
Moreover, we wanted to see if postponing the recovery period during three trials with a single recovery phase would prolong the time to exhaustion at the end of the trial. That is, would more extensive use of D’ in the first bout promote faster D’ reconstitution ahead of the second bout?
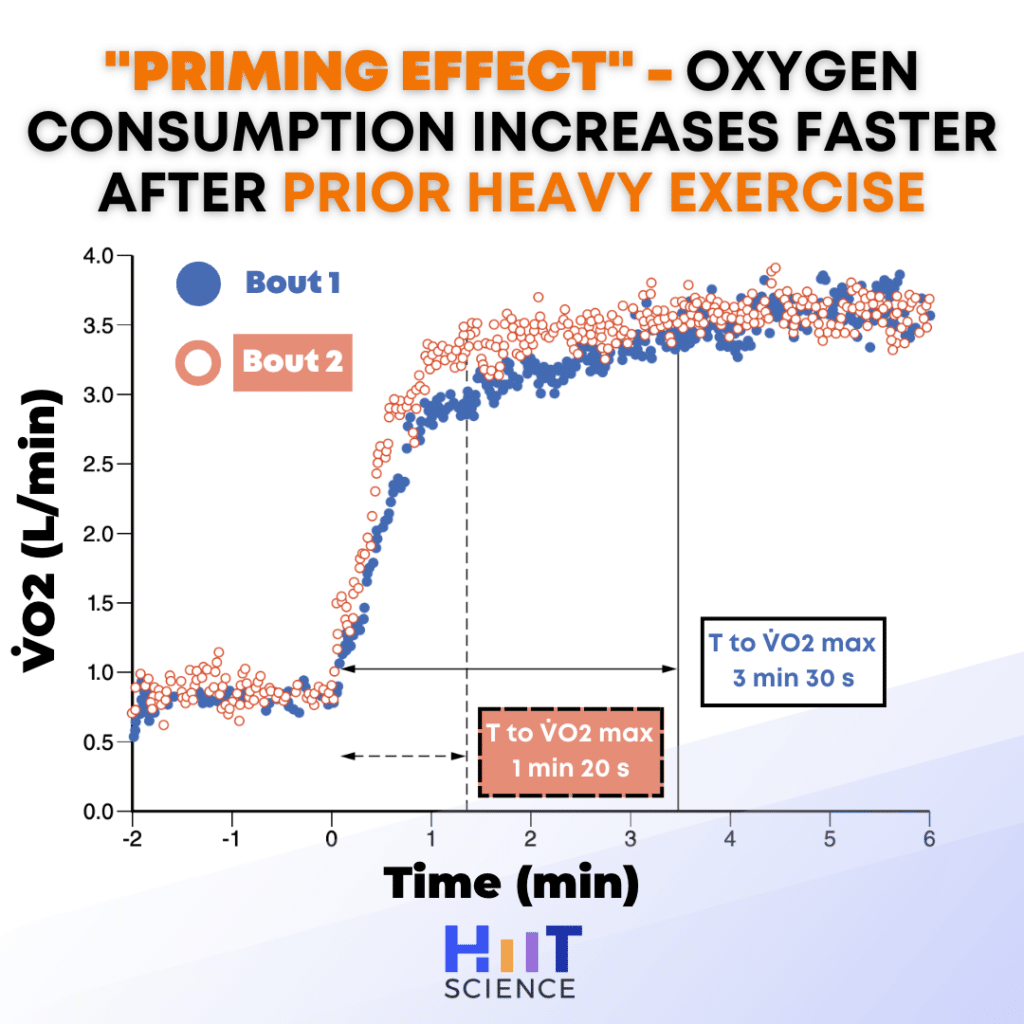
“A method in the madness” – testing battery
Nine well-trained runners completed four separate tests (Fig. 5): an incremental test, velocity-duration trials, D’ reconstitution trials, and HIIT test.
Every test was conducted on a running track, and the running pace was set by an operator with the help of markers placed every 20 meters and a device emitting an acoustic signal at the time at which the subject should cover the distance between the markers to match the individually prescribed pace. In other words, each “beep” corresponded to the time in which 20 m should have been covered if one ran at the predetermined speed (e.g., at 2.7 m/s a beep every 7.4 s). The test ended when the runner failed to cover two consecutive markers just before or at the sound of the signal.
The maximal running step-incremental test was needed to measure VO2max and maximal aerobic speed (vVO2max). Knowing these parameters, we made participants run three to five exhaustive trials at different speeds (between 90 and 110% of vVO2max) to determine CV and D’ (Poole et al. 2016; Jones and Vanhatalo 2017; Jones et al. 2019). All the tests had to last a minimum of ~ 2 and a maximum of ~ 15 min to be considered valid (Jones and Vanhatalo 2017). Then, the CV and D’ were calculated using two methods: The distance-time method and the 1/time method (Clarke and Skiba 2013).
Subsequently, to investigate the reconstitution characteristics of D’, the participants performed three trials comprised of one high-intensity bout followed by 2 minutes of active recovery followed by a second high-intensity bout continued until exhaustion. The first bout was used to simulate three different involvement situations of D’: large usage (3 minutes), little usage (30 seconds), and complete usage (run to exhaustion). The running speed was set to 120% of the CV to a velocity supposed that would lead to exhaustion after roughly 6 minutes (Jones et al. 2010). The recovery phase served to the reconstitution of D’, speed was set at 66% of the CV. The second high-intensity bout’s purpose was to consume every bit of D’ reconstituted during the recovery phase. Hence, the longer the second bout was, the more D’ must have been reconstituted.
Finally, we tested the HIIT protocol which used the same intensities as the D’ reconstitution trials. In this case, we tested three different protocols:
- Long intervals (LIHIIT): 3 min at high intensity and 2 min at low intensity repeated until volitional exhaustion of the subject (Fig. 5F).
- High-intensity decremental interval training (HIDIT): 3 min at high intensity and 2 min at low; 2 min at high and 1 min and 20 s at low; 1 min at high and 40 s at low; 45 s at high and 30 s at low; and finally, 30 s at high and 20 s at low intensity repeated until volitional exhaustion of the subject (Fig. 3; Fig. 5G).
- Short intervals (SIHIIT): 30 s at high intensity and 20 s at low intensity until volitional exhaustion of the subject (Fig. 5H)
The purpose was to measure the time spent above 90% of VO2max for every HIIT protocol.
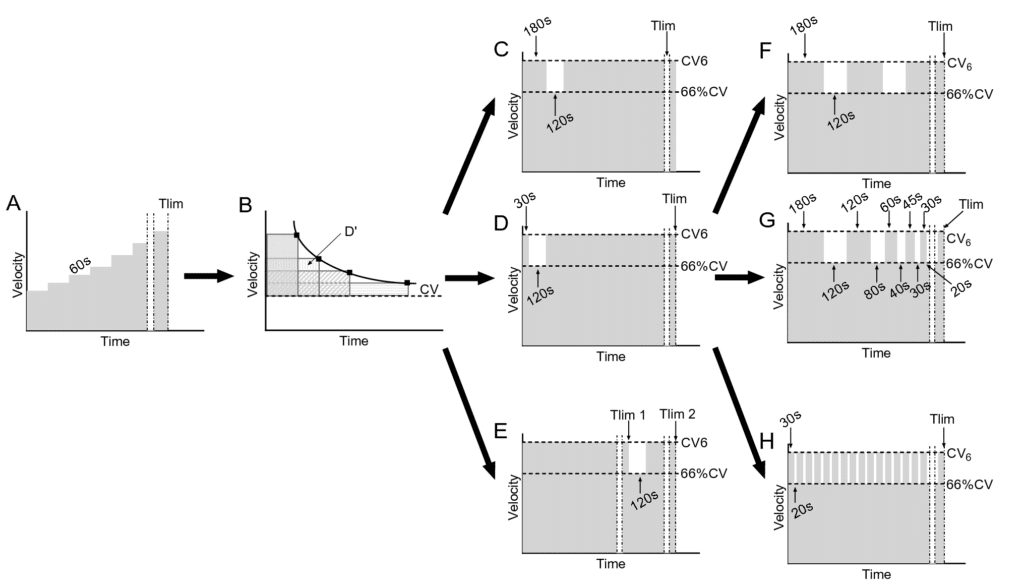
Figure 5. Study design. Incremental test (A) and velocity-duration trials (B) (velocity-duration trials’ intensities were used for the following sessions). Three test trials (C, D, and E) were then performed to investigate the reconstitution of D’ after three different durations of exercise at 120% CV (3 min, 30 s, and exhaustion). The three HIIT protocols: LIHIIT, HIDIT, and SIHIIT (F, G, and H) were investigated for T90%VO2max and Tlim.
The more D’ you put in, the more VO2 you “get out” – Results
Following all of the exercise tests we came away with two main findings.
First, the total time spent at high intensity during the reconstitution D’ trials was on average 308 ± 44 s, 388 ± 48 s, and 464 ± 67 s for the 30 seconds, 3 minutes, and exhaustion bout, respectively, which was significantly different between each trial (Fig. 6). Reflecting the bi-exponential characteristics of D’ (W’) reconstitution. That is, the larger the D’ depletion is, the faster it is recovered.
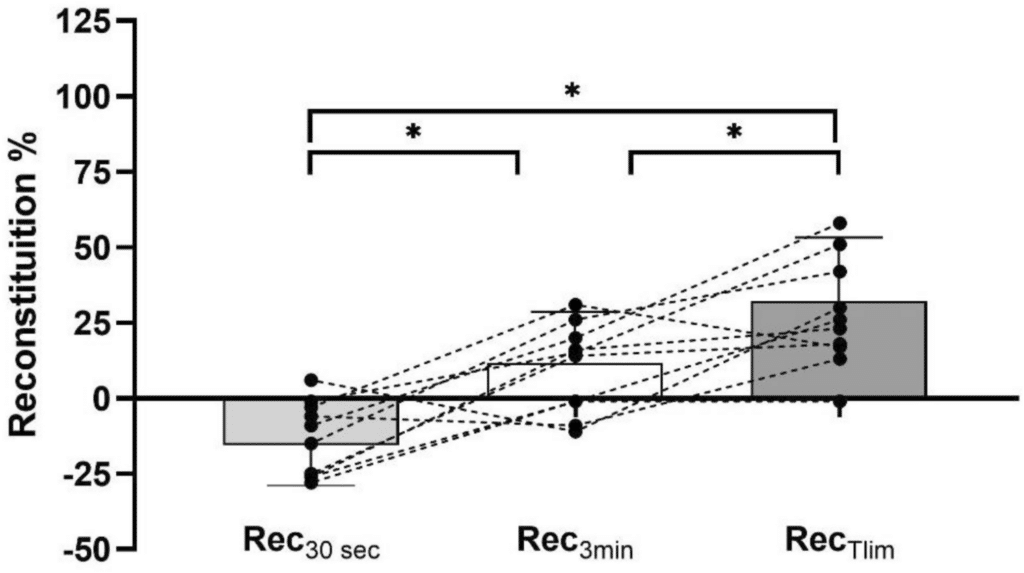
Figure 6. Reconstitution of D’ as a percentage restored after 2 minutes of recovery phase at 66% of CV. The calculation was done assuming that subjects’ theoretical D’, calculated with either 1/time or distance/time method, would be entirely consumed after 6 minutes if ran at CV6. By calculating the ratio of theoretical D’ consumption during a CV6, we found how much D’ would’ve been consumed every second, so by multiplying that number by the actual time spent during high-intensity bouts during the trials we found how much D’ had been used. Next, we subtracted the theoretical D’ from the actual one measured during the trials to find how much D’ was restored after the recovery phase. Since theoretical D’ is the maximum level (100%), D’ recovered in 2 minutes would be a small percentage of it. Doing a simple proportion, we found the right percentage of D’ restored.
Secondly, the differences between the HIIT protocols were observed in the time to exhaustion (Tlim) (Fig. 7A), and the time above 90% of VO2max (T90%VO2max) (Fig. 7B). In particular, the Tlim of HIDIT was significantly larger than those of the LIHIIT and SIHIIT protocols (998 ± 129 s, 673 ± 115 s, and 675 ± 116 s, respectively). Likewise, HIDIT elicited a significantly larger duration of work above 90% of VO2max than the other two HIIT protocols (579 ± 219 s HIDIT, 349 ± 111 s LIHIIT, and 167 ± 188 s SIHIIT).
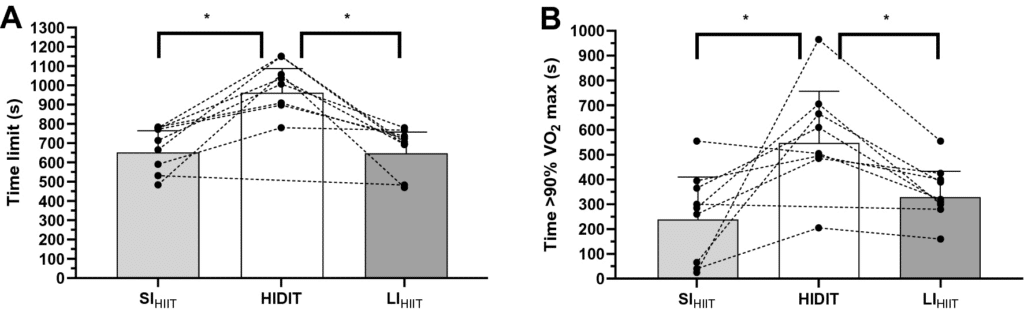
Figure 7. Time limit (A) and Time >90%VO2max (B) during HIIT protocols.
A decreasing stairway to VO2max – Discussion
In our study, we observed that the reconstitution of D’ benefited from a postponed recovery interval and this allowed a longer second bout of high-intensity exercise. Moreover, HIDIT has proven to allow longer times above 90%VO2max (T90%VO2max) and Tlim compared to LIHIIT and SIHIIT, despite having the same mean velocity, duration, and ratio of the high-to-low intensity of the intervals.
The exponential behaviour of W’ (the cycling equivalent of D’) was seen in several studies performed on a cycle ergometer (Ferguson et al. 2010; Skiba et al. 2014; Caen et al. 2019; Sreedhara et al. 2020; do Nascimento Salvador et al. 2021; Lievens et al. 2021; Caen et al. 2021; Chorley et al. 2021). With our work, we investigated such behaviour in running performance.
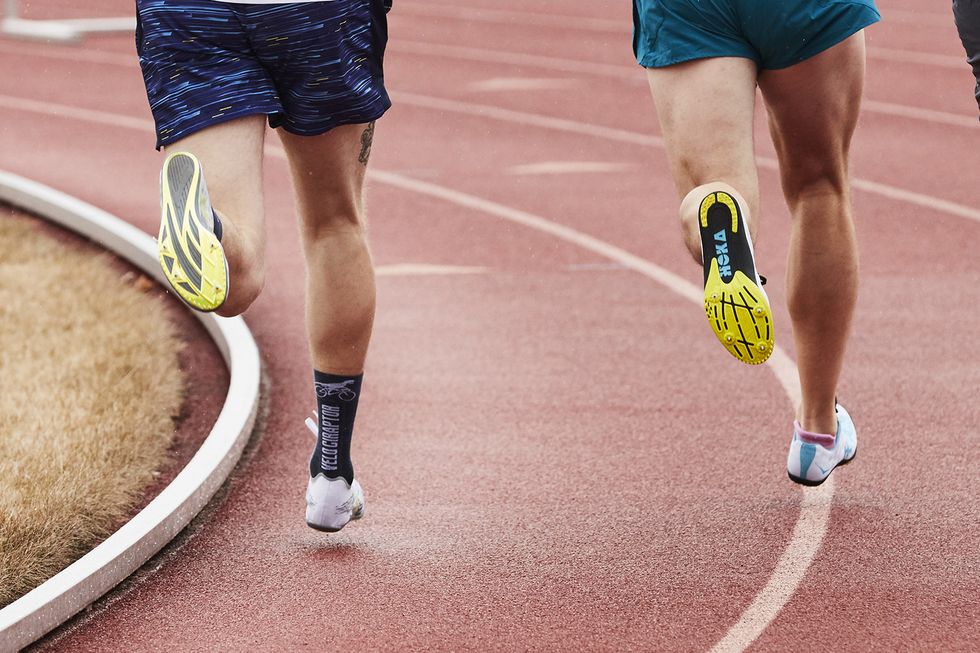
We previously observed that in cyclists HIDIT can increase the time above 90%VO2max compared to HIIT protocols with short or long intervals (Vaccari et al. 2020). This effect could be attributed to the priming effect of longer intervals at the start combined with the more efficient usage and reconstitution rate of D’. However, the results of the cycling study were slightly different since HIDIT did not significantly increase Tlim. Contrastingly, in this year’s study, we observed that both T90%VO2max and Tlim are extended in a HIDIT running session. This disparity could be explained by the more profound development of VO2 slow component and hence fatigue during high-intensity cycling compared to running (Carter et al. 2000).
Practical Applications
In adult active males, training protocols starting with long intervals followed by short intervals could be useful to accumulate time close to VO2max and consequently improve it.
An important aspect was that HIDIT would not seem physiologically more fatiguing than other classical protocols, so the benefits should not be accompanied by more fatigue. Thus, it could be used in a general preparation phase in the same way as traditional HIIT protocols and could be another tool in the coaches’ toolbox to promote stimuli variation.
About the author – Jacopo Stafuzza
I am a research fellow at the University of Udine, working on a project promoting physical exercise as a tool for preventing and treating chronic diseases. I never considered myself as an athlete. During my childhood and adolescence, I tried a lot of sports but could not find the right one for me. Only during my mid-20s, I found rock climbing, which happened to be a love at “first crimp”. Being a very curious person, I developed a passion for training and improving athletic performance. I decided to go to the university to study Exercise and Sports Science. I wanted to better understand human movement and try to find answers to my questions (Fool! I ended up with more questions than answers). I graduated from the University of Udine in Sports Science with a master’s thesis on the effect of different HIIT training protocols on running performance. Even though I am not a runner, my curious nature spurred a great passion and dedication to this project.
References
Buchheit, M., & Laursen, P. B. (2013). High-intensity interval training, solutions to the programming puzzle: Part I: cardiopulmonary emphasis. Sports Medicine, 43(5), 313–338. https://doi.org/10.1007/s40279-013-0029-x
Burnley, M., Doust, J. H., Ball, D., & Jones, A. M. (2002). Effects of prior heavy exercise on VO2 kinetics during heavy exercise are related to changes in muscle activity. Journal of Applied Physiology, 93(1), 167–174. https://doi.org/10.1152/japplphysiol.01217.2001
Caen, K., Bourgois, G., Dauwe, C., Blancquaert, L., Vermeire, K., Lievens, E., Van Dorpe, J., Derave, W., Bourgois, J. G., Pringels, L., & Boone, J. (2021). W′ recovery kinetics after exhaustion: A two-phase exponential process influenced by aerobic fitness. Medicine & Science in Sports & Exercise, 53(9), 1911–1921. https://doi.org/10.1249/mss.0000000000002673
Caen, K., Bourgois, J. G., Bourgois, G., Van der Stede, T., Vermeire, K., & Boone, J. (2019). The reconstitution of W′ depends on both work and recovery characteristics. Medicine & Science in Sports & Exercise, 51(8), 1745–1751. https://doi.org/10.1249/mss.0000000000001968
Carter, H., Jones, A. M., Barstow, T. J., Burnley, M., Williams, C. A., & Doust, J. H. (2000). Oxygen uptake kinetics in treadmill running and cycle ergometry: A comparison. Journal of Applied Physiology, 89(3), 899–907. https://doi.org/10.1152/jappl.2000.89.3.899
Chorley, A., Bott, R. P., Marwood, S., & Lamb, K. L. (2021). Bi-exponential modelling of W′ reconstitution kinetics in trained cyclists. European Journal of Applied Physiology, 122(3), 677–689. https://doi.org/10.1007/s00421-021-04874-3
Clarke, D. C., & Skiba, P. F. (2013). Rationale and resources for teaching the mathematical modeling of Athletic Training and performance. Advances in Physiology Education, 37(2), 134–152. https://doi.org/10.1152/advan.00078.2011
do Nascimento Salvador, P. C., de Lucas, R. D., Schäfer, L., Guglielmo, L. G., Grassi, B., & Denadai, B. S. (2021). Modeling the depletion and reconstitution of W′: Effects of prior exercise on cycling tolerance. Respiratory Physiology & Neurobiology, 285, 103590. https://doi.org/10.1016/j.resp.2020.103590
Ferguson, C., Rossiter, H. B., Whipp, B. J., Cathcart, A. J., Murgatroyd, S. R., & Ward, S. A. (2010). Effect of recovery duration from prior exhaustive exercise on the parameters of the power-duration relationship. Journal of Applied Physiology, 108(4), 866–874. https://doi.org/10.1152/japplphysiol.91425.2008
Hill, A. V., & Lupton, H. (1923). Muscular exercise, lactic acid, and the supply and utilization of oxygen. QJM, os-16(62), 135–171. https://doi.org/10.1093/qjmed/os-16.62.135
Jones, A. M., Wilkerson, D. P., Vanhatalo, A., & Burnley, M. (2008). Influence of pacing strategy on O2 uptake and exercise tolerance. Scandinavian Journal of Medicine & Science in Sports, 18(5), 615–626. https://doi.org/10.1111/j.1600-0838.2007.00725.x
Jones, Andrew M., & Vanhatalo, A. (2017). The ‘critical power’ concept: Applications to sports performance with a focus on intermittent high-intensity exercise. Sports Medicine, 47(S1), 65–78. https://doi.org/10.1007/s40279-017-0688-0
Jones, Andrew M., Burnley, M., Black, M. I., Poole, D. C., & Vanhatalo, A. (2019). The maximal metabolic steady state: Redefining the ‘Gold Standard.’ Physiological Reports, 7(10), e14098. https://doi.org/10.14814/phy2.14098
Lievens, M., Caen, K., Bourgois, J. G., Vermeire, K., & Boone, J. (2021). W′ reconstitution accelerates more with decreasing intensity in the heavy- versus the moderate-intensity domain. Medicine & Science in Sports & Exercise, 53(6), 1276–1284. https://doi.org/10.1249/mss.0000000000002574
Midgley, A. W., & Mc Naughton, L. R. (2006). Time at or near VO2max during continuous and intermittent running. A review with special reference to considerations for the optimisation of training protocols to elicit the longest time at or near VO2max. J Sports Med Phys Fitness, 46(1), 1–14.
Millet, G. P., Candau, R., Fattori, P., Bignet, F., & Varray, A. (2003). Responses to different intermittent runs at velocity associated with VO2max. Canadian Journal of Applied Physiology, 28(3), 410–423. https://doi.org/10.1139/h03-030
Nixon, R. J., Kranen, S. H., Vanhatalo, A., & Jones, A. M. (2021). Steady-state V̇O2 above MLSS: Evidence that critical speed better represents maximal metabolic steady state in well-trained runners. European Journal of Applied Physiology, 121(11), 3133–3144. https://doi.org/10.1007/s00421-021-04780-8
Poole, D. C., Burnley, M., Vanhatalo, A., Rossiter, H. B., & Jones, A. M. (2016). Critical power. Medicine & Science in Sports & Exercise, 48(11), 2320–2334. https://doi.org/10.1249/mss.0000000000000939
Skiba, P. F., Jackman, S., Clarke, D., Vanhatalo, A., & Jones, A. M. (2014). Effect of work and recovery durations on W′ reconstitution during intermittent exercise. Medicine & Science in Sports & Exercise, 46(7), 1433–1440. https://doi.org/10.1249/mss.0000000000000226
Sreedhara, V. S., Ashtiani, F., Mocko, G. M., Vahidi, A., & Hutchinson, R. E. (2020). Modeling the recovery of W′ in the moderate to heavy exercise intensity domain. Medicine & Science in Sports & Exercise, 52(12), 2646–2654. https://doi.org/10.1249/mss.0000000000002425
Vaccari, F., Giovanelli, N., & Lazzer, S. (2020). High-intensity decreasing interval training (HIDIT) increases time above 90% VO2peak. European Journal of Applied Physiology, 120(11), 2397–2405. https://doi.org/10.1007/s00421-020-04463-w