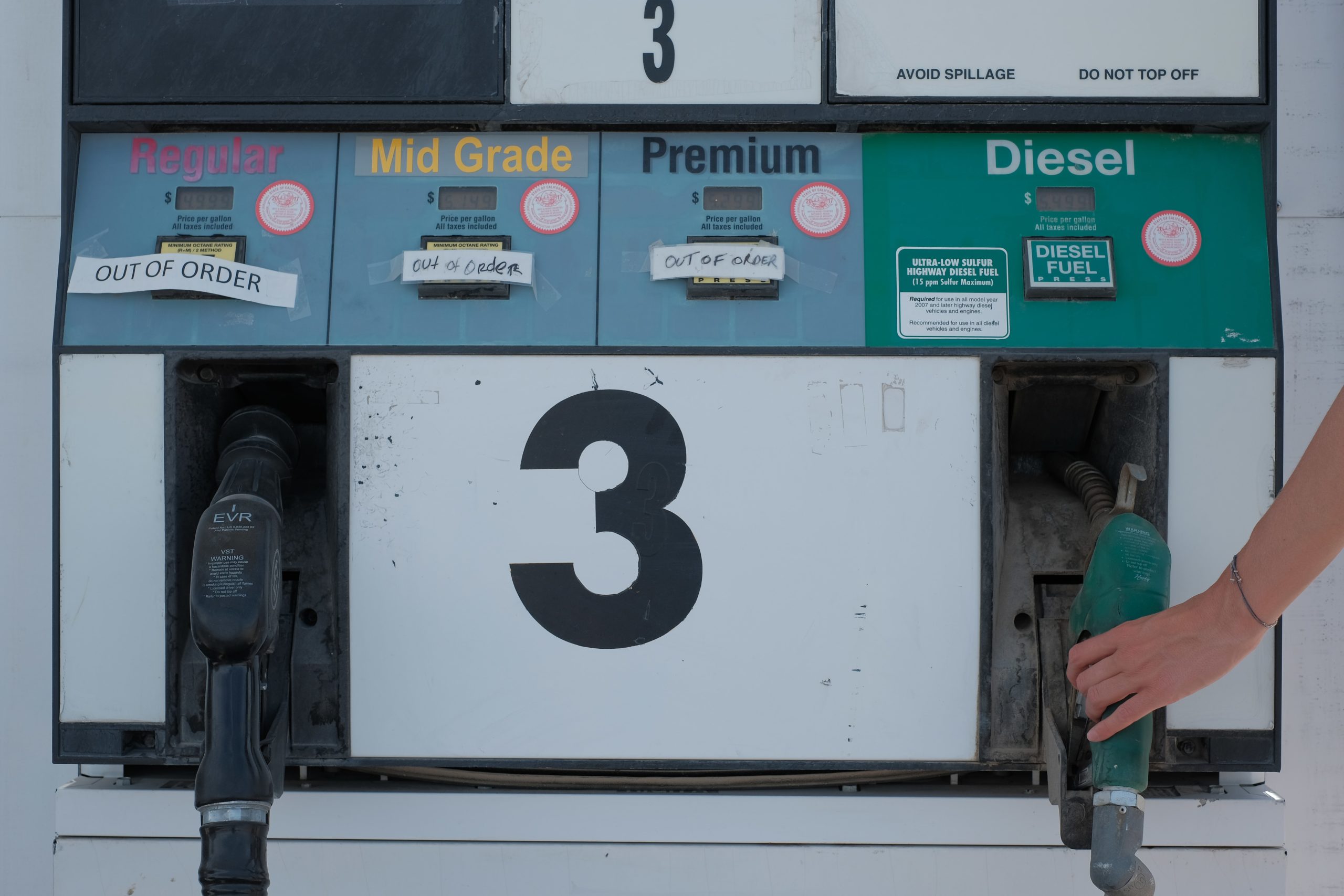
Aim
Using a novel over-ground energetics model, the purpose of this research was to evaluate the mechanical and energetic cost of two commonly-used HSR drills in elite team sports: box-to-box and tempo runs. We also wanted to provide insight into the importance of individualisation when it comes to programming these specific training drills. Therefore, we modelled the anticipated responses of four simulated players with distinct locomotor profiles.Design
We used a case-study modelling design of over-ground running energetics on simulated athlete profiles to determine acute session responses. Four athlete profiles were constructed: 1) Fit and Fast; 2) Fit and Slow; 3) Less Fit and Fast; 4) Less Fit and Slow (Table 1). Each athlete underwent fixed HIIT (all together, same running distance), individualised HIIT (100% velocity achieved at end of 30-15 Intermittent Fitness Test; VIFT) and tempo runs on separate occasions. Sessions were volume-matched at 18 repetitions. Raw GPS velocity data were modelled to over-ground mechanical power using an energetics model. Responses to sessions were then evaluated accordingly.For more details about the methods, you can check the full paper in Sport Performance & Scientific Reports.
Figure 1. Detailed running prescriptions for the four locomotor player profiles when programming fixed HIIT box-to-box runs, individualised HIIT box-to-box runs or tempo box-to-box runs.
Table 1. Selected player profiles.Notes. MSS = maximal sprinting speed; MAS = maximal aerobic speed; ASR = anaerobic speed reserve; VIFT= velocity achieved upon termination of the 30-15 Intermittent Fitness Test; CS = critical speed; D´ = finite distance capacity above CS; CP = critical power; W´ = finite work capacity above CP.
Results
The three mechanical and energetic simulations for the four locomotor player profiles are shown in Figure 2 (fixed HIIT box-to-box runs), 3 (individualised HIIT box-to-box runs), and 4 (tempo box-to-box runs). External load responses All three box-to-box running drills allowed players to cover between 1200-1300 m of total distance and 500-800 m in the HSR (> 19.8 km/h) zones. Internal load responses There were very large between-player profile differences in W´ depletion with the fixed HIIT box-to-box runs (Figure 2 and Table 2), i.e., with Fit & Fast and Less Fit & Slow players finishing with 65% and 27% of their W´BAL, respectively. In contrast, with the individualised HIIT prescription, all player profiles finished within the same level of W´ depletion (approx 40% for all, Figure 3 and Table 2).Figure 2. Fixed HIIT box-to-box consisting of 72 m runs in 13 s, interspersed by 17 s for series 1 (10 repetitions) and series 2 (8 repetitions), with 2 min recovery between series. External mechanical power output (P) and balance of remaining W´ (W´BAL), expressed in relative %, is displayed for all four athlete profiles. Note how despite the fixed running speed (19.9 km/h), there exist differences in over-ground mechanical power output accounting for the influence of body mass and stature (i.e. varied player profile) on work done during each work interval. When P is > CP, W´ is expended; when P is < CP, W´ is reconstituted, such that the balance of W´ remaining (W´BAL) was quantified across the HIIT session. Note also the influence of higher critical power (i.e. aerobic ‘fitness’) on the between-series recovery kinetics of W´. Fixed HIIT produced large disparity in inter-individual responses.
Figure 3. Individualised HIIT box-to-box at 100% VIFT. Runs in 13 s, interspersed by 17 s for series 1 (10 repetitions) and series 2 (8 repetitions), with 2 min recovery between series. For each athlete profile, speed (100% VIFT) was modelled into external mechanical power output (P) using the over-ground running energetics model. Balance of remaining W´ (W´BAL), expressed in relative %, is displayed for all four athlete profiles. When P is > CP, W´ is expended; when P is < CP, W´ is reconstituted, such that the balance of W´ remaining (W´BAL) was quantified across the HIIT session. Individualised HIIT achieved a homogeneous internal response for all four athlete profiles.
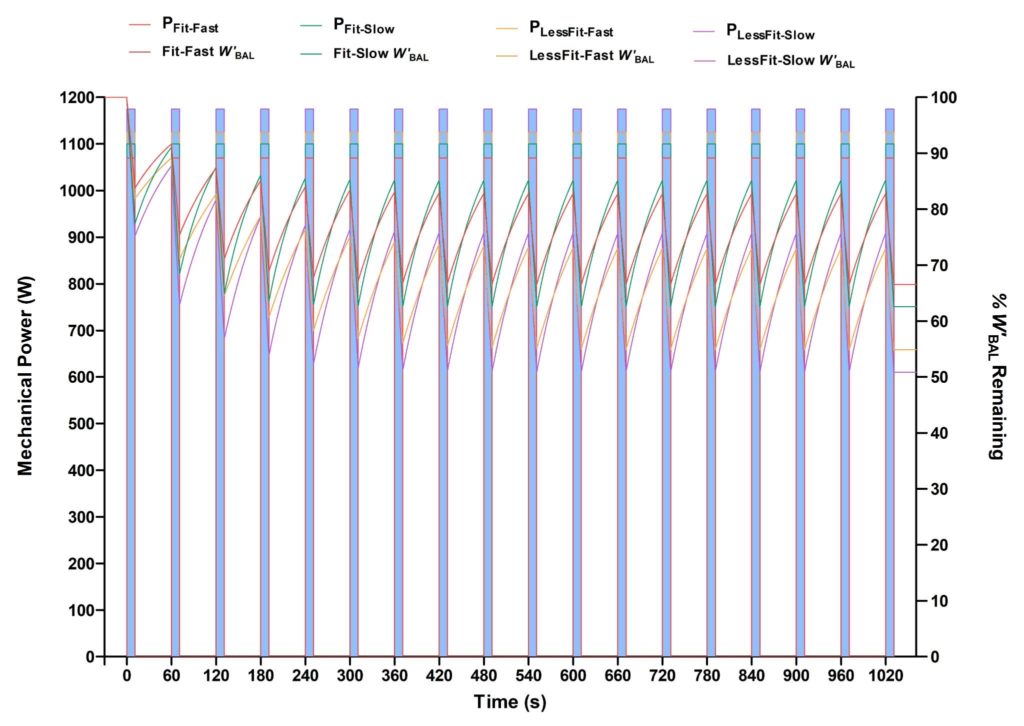
Figure 4. Tempo box-to-box runs consisting of 72 m runs in 11 s, interspersed by 49 s for a total volume of 18 repetitions. External mechanical power output (P) and balance of remaining W´ (W´BAL), expressed in relative %, is displayed for all four athlete profiles. Note how despite the fixed running speed (23.6 km/h), there exist differences in over-ground mechanical power output accounting for the influence of body mass and stature (i.e. varied player profile) on work done during each work interval. Note also the preservation of W´BAL (> 50%) and reduction of metabolic stress induced across all four profiles, with the accompanying larger mechanical power outputs likely eliciting greater neuromuscular load.
Table 2. Balance of remaining W´ (W´BAL) expressed in relative % for all four player profiles at the end of each series for each box-to-box drill.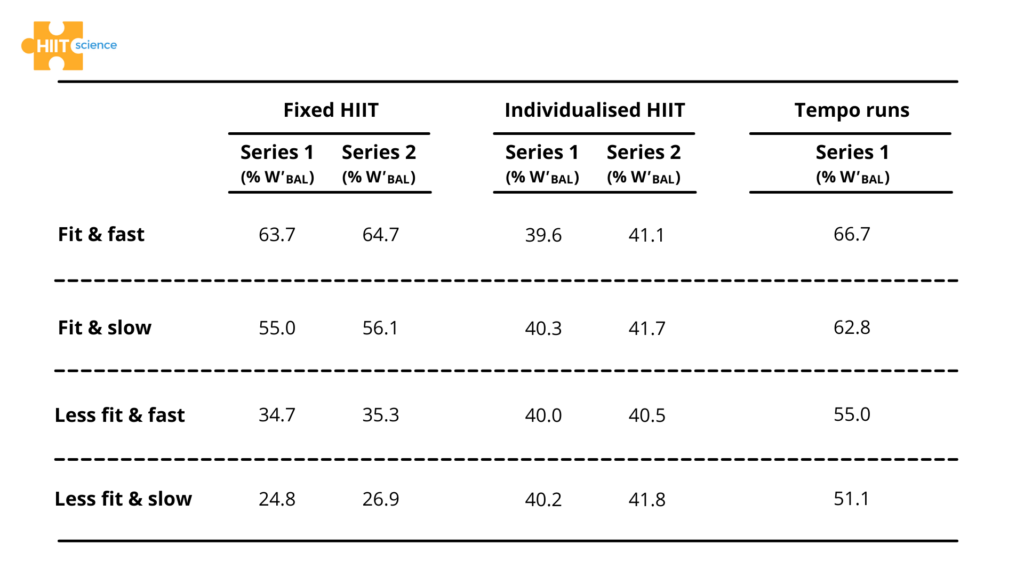
Discussion
The first observation from the present study was that when players with different locomotor profiles performed similar, fixed HIIT box-to-box runs, they presented with very large differences in internal energetic responses. While some finished their series with W´ almost unaffected, for others, it approached near-severe depletion (Figure 2 and Table 2). When individualising the distance of the runs based on players’ individual locomotor profiles (Figure 3 and Table 2), however, the magnitude of W´ depletion and the likely associated metabolic demands and neuromuscular fatigue were very similar.This lends support to the principle of exercise intensity individualisation to induce homogeneous physiological responses and, in turn, a more precise and efficient training stimulus (Buchheit, 2008; Laursen & Buchheit, 2019).While this is regularly seen in today’s football (mainly after matches as part of a substitute’s compensation workout), the present results question this current fixed practice that consists of having all players run together during box-to-box drills.
The direct consequences of not individualising running drills are various, with some players receiving insufficient metabolic load, and others completing the drills in an advanced state of both metabolic stress and neuromuscular fatigue.In short, while the individualised approach allows practitioners to target specific biological systems, i.e., both the aerobic and anaerobic metabolic systems, and neuromuscular system consecutive to the 500-800 m of HSR (considered as Type 4 HIIT format following the HIIT Science classification) (Laursen & Buchheit, 2019), it remains unclear what the contribution of these biologic systems is when using the fixed box-to-box approach (and which HIIT Type they fit in). This complicates, rather than helps to solve the training plan, since the aim is generally to manage the individual within the team, avoid excessive differences in load (e.g., starters vs. substitutes) and compensate their load accordingly at the individual level.
The modelling of responses to the Tempo box-to-box runs (Figure 4) showed that this method allows preservation of the W´ for all locomotor profiles (i.e., >50% for all, Table 2), and suggests that these types of drills lack sufficient metabolic stress to be classified as HIIT.In fact, HIIT is generally defined as exercise eliciting at least 90% of maximal oxygen uptake (Midgley & Mc Naughton, 2006), which often occurs during intermittent exercise with substantial concomitant W´ depletion (Chidnok et al., 2012; Skiba et al., 2012).
Therefore, Tempo runs should be used to accumulate HSR, but not necessarily as a metabolic conditioning tool.If we continue to follow the HIIT Science classification, that makes Tempo runs in fact likely Type 6 (high neuromuscular load, but low-to-moderate aerobic and anaerobic response). Of interest, since both the HIIT and Tempo run drills included 18 repetitions, the total distance ran at high speed (above 19.8 km/h) was similar (500-800m); in reality however, the peak speeds reached were likely higher for the Tempo (24-26 km/h) compared with the HIIT (20-24 km/h) runs. Overall, this suggests that Tempo runs could be used as an alternative to the other box-to-box drills when accumulation of HSR is required, but without a metabolic conditioning objective (rehab players, congested fixtures, etc.). Finally, it is worth commenting on the timing of implementation of these box-to-box running drills (e.g. pre-, intra- or post-session) (Buchheit, 2019b). The internal energetic responses (Table 2) were modelled with all four profiles starting at 100% W´BAL, which is consistent with a scenario where drills are prescribed either at the beginning of the session or as a post-match HSR top-up for benched players (i.e. high degree of ‘freshness’). The actual degree of metabolic perturbation reported (% W´BAL depletion) may therefore be greater for HIIT drills programmed later in the session, with players having undergone prior W´ depletion.
Practical applications
- Practitioners choosing to have all players run together during fixed box-to-box runs, irrespective of their metabolic profile, expose them to varying metabolic responses and neuromuscular loads. This is likely to be problematic at many levels, since it does not guarantee optimal player development, adding unnecessary fatigue to some players.
- Prescribing individualised HIIT using a players’ locomotor profile and/or the VIFT allows a tight individualisation of exercise intensity, and in turn, provides a homogeneous and optimal metabolic and neuromuscular load to all players.
- Tempo runs should be used as a tool to accumulate HSR, and as an alternative to HIIT in specific situations when metabolic conditioning is not the objective.
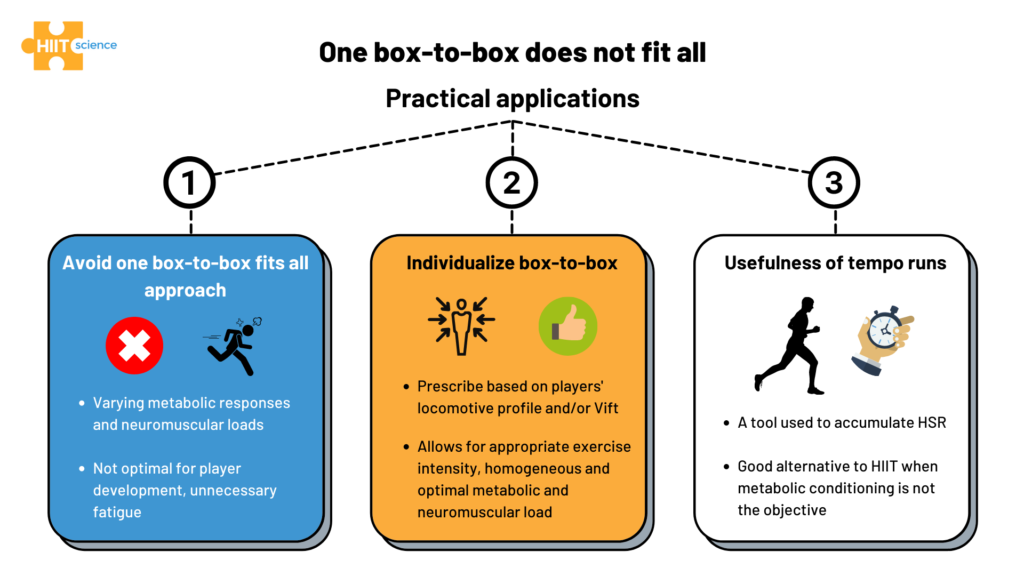