Background
The emergence of continuous glucose monitoring (CGM) devices has enabled deeper insight into the temporal dynamics of blood glucose concentration under real life settings (1). Compared with traditional finger prick samples, which are invasive and obstructive to natural activity, CGMs allow non-invasive high frequency sampling. These CGM devices only need to be applied once (usually on the back of the arm), before being used for several days without disturbing daily practices, including feeding, sleeping, sport and recovery. So far, these devices have been mainly used by diabetic populations (2), but as the technology becomes more accurate, less invasive, and less expensive, their use has increased in other populations and especially in healthy individuals, including athletes. Therefore, the inclusion of CGM monitoring, as with GPS, heart rate and power meter monitoring, could help to optimize nutritional strategies for athletes before and during exercise, and in turn, improve athletes’ performance. However, to date, the validity of these new systems at rest or during exercise has been mostly only assessed in diabetics patients (2). Evidence regarding its relevance in an athletic population, although described in blog posts (3), is still limited in the scientific literature. Moreover, the ability of such devices to detect potential glucose fluctuations due to different nutritional intakes and dietary practices needs to be confirmed. In this blog, we summarise the findings of our validity study recently accepted for publication in the International Journal of Sports Performance and Physiology.What did we do?
We recruited eight non-diabetic recreational athletes (5 females, 3 males; age: 30.8 ± 9.5 years; height: 173.6 ± 6.6 cm; body mass: 70.3 ± 8.1 kg) who regularly participate in running and resistance-based training (8±2 hours per week). A concurrent validity study design was employed to assess the validity of a CGM system against finger prick measures, which was considered as the reference method. The protocol, as well as the HIIT session used in this study, are displayed in Figure 1. Figure 1. Schematic representation of the study design (upper panel) and the HIIT session (lower panel).
What did we find?
For a comprehensive view of the results, you can read the full paper here.Validity during the HIIT session
We observed a higher bias during exercise compared with the other periods (Figure 2). Reasons that may contribute to the reduced validity of the CGM device in this context include microcirculation perturbations as a result of movements around or within the insertion area, increases in body temperature, and rapid fluxes in glucose levels during exercise (4). Regarding the likely physiological time lag of glucose transport between blood and interstitial fluid compartments (finger pricks measured changes faster Post-Breakfast than the CGM device), it should be noted that this finding might not have accounted for the observed difference in accuracy as the pattern is not only delayed but varies with time and condition (Figure 2). Indeed, while hypoglycemia was observed with the finger prick measurement immediately at the start of exercise (which was the expected physiological response), the CGM showed an increased blood glucose response. This discrepancy suggests that the CGM device was unable to detect a potential hypoglycemia observed at the onset of exercise, and could therefore not be used to assess strategies aiming at preventing this phenomenon in practice. It is worth mentioning that a trend for better agreement was observed toward the end of the exercise periods. If the duration of the exercise also affects the accuracy of CGM, it may mean that the device is not currently suitable for use in sport, including short and intermittent exercise durations. However, its use could perhaps be considered in longer events such as cycling, trail running, or triathlon (see example response here). This potential better accuracy toward longer exercise duration highlights the need to conduct further research involving 1) longer exercise duration, 2) nutritional intake during long endurance races, 3) various exercise modalities, 4) different intensities and 5) different levels of fat adaptation (very fat-adapted athletes may have chronically lower blood glucose responses during exercise [3]). Figure 2. Continuous glucose monitoring (CGM) and finger prick measures during each standardized condition, when ingesting a carbohydrate- (upper) and protein- (lower) oriented breakfasts, with the 2 days of each breakfast condition pooled for each participant (n = 2 x 8 for each curve). Data are presented as mean (SE).
Effect of Nutrition
Similar results were observed for the carbohydrate and protein feedings, suggesting that the CGM validity was not affected by the breakfast content during non-exercise periods (i.e. Post-Breakfast, Pre-Exercise, Post-Exercise) (Figure 2). Consequently, the use of this CGM device could be considered by practitioners willing to monitor glycemic responses before and after competition or training, to ensure the efficacy of the nutritional strategies employed. However, during the exercise period, the CGM accuracy was modulated by breakfast content. For example, finger prick measures indicated a hypoglycemic state whereas CGM measures were within the normal range suggesting that CGM failed to detect the hypoglycemia occurring during exercise after the CHO-rich breakfast. It is well known there is a rapid drop of blood glucose concentration at the onset of exercise, due to an increased glucose uptake by exercising muscles (5). This physiological mechanism could explain why the sensor lacks sensitivity to rapid changes in glucose concentration, as observed in the present study. As it stands, if practitioners want to monitor blood glucose during high-intensity intermittent exercise, they may want to consider other devices other than CGM (e.g. finger prick).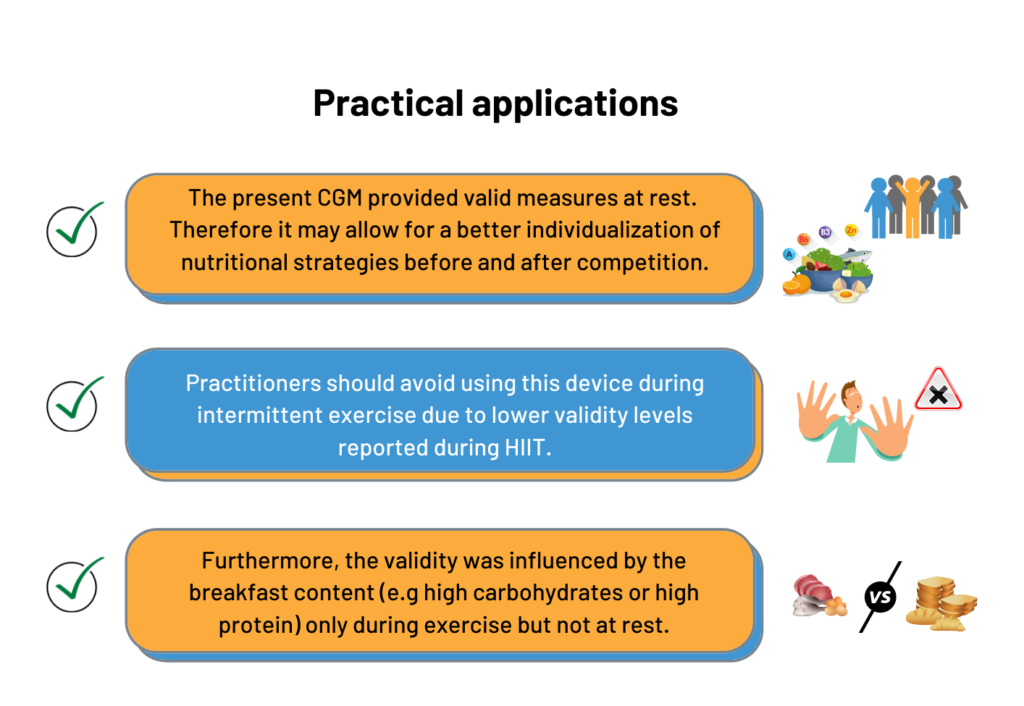
Reference
- Hall H, Perelman D, Breschi A, et al. Glucotypes reveal new patterns of glucose dysregulation. PLoS Biol. 2018;16(7):1-23. doi:10.1371/journal.pbio.2005143
- Biagi L, Bertachi A, Quirós C, et al. Accuracy of continuous glucose monitoring before, during, and after aerobic and anaerobic exercise in patients with type 1 diabetes mellitus. Biosensors. 2018;8(1):1-8. doi:10.3390/bios8010022.
- Laursen, P.B. The “Peter Attia” Approach to Dieting for Endurance Athletes (Part One). TrainingPeaks Blog Post. 2017.
- Kumareswaran K, Elleri D, Allen JM, et al. Accuracy of continuous glucose monitoring during exercise in type 1 diabetes pregnancy. Diabetes Technol Ther. 2013;15(3):223-229. doi:10.1089/dia.2012.0292.
- Richter EA, Hargreaves M. Exercise, GLUT4, and skeletal muscle glucose uptake. Physiol Rev. 2013;93(3):993-1017. doi:10.1152/physrev.00038.2012.